CATIONIC PEPTIDES: A NEW HOPE
(August 2004)
The discovery of penicillin in 1929 by Alexander Fleming was a milestone in modern medicine [1]. In 1943, the industrial-scale production of penicillin enabled its widespread use and ushered in the beginning of the “antibiotic era.” The availability of antibiotics has had profound effects on human health and has contributed to an eight-year increase in the average human lifespan [2]. The availability of antibiotics has allowed for the successful treatment of many bacterial infections as well as the ability to perform invasive medical procedures including surgery and chemotherapy [3]. Throughout the years, however, many bacterial strains have evolved ways to adapt or become resistant to the currently available antibiotics [3]. An example is Staphylococcus aureus of which more than 95% of the strains are resistant to the antibiotic penicillin [4]. This is problematic since very few new families of antibiotics have been discovered in the past 30 years [3]. One of the reasons that antibiotic resistance is so widespread is their pervasive use in animal feeds to prevent infections and promote growth [5]. This has encouraged spontaneous mutation of the targets of anti-bacterial drugs as well as the exchange of plasmids encoding antibiotic resistant genes4. There are many mechanisms by which bacteria evade antibiotic treatment:[4]
- reduced drug uptake
- active pumping of drugs out of the cell
- enzymatic alteration of the antibiotic
- modification of targets
- drug sequestering by protein binding
- overproduction of the target
- metabolic bypass of the targeted pathway
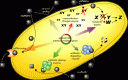
Antibiotics are an estimated 7-22 billion dollar a year industry and the third largest class of drug sold [4]. There are currently over 150 antimicrobial drugs that have been approved for use in the United States as well as approximately 27 currently in clinical development [4]. Many of these new antibiotics in development will not be approved by the US Food and Drug Administration as only a handful have been approved in the last 10 years [4]. Most of the drugs in development are analogs of earlier antibiotics which work on a select number of bacterial targets [4]. There will be an increasing demand for novel drugs to combat bacterial infections in the near future [6].
One approach to this problem of resistance to current antibiotics is to look to “evolutionary ancient weapons” [7]. All multicellular organisms have evolved in the presence of a plethora of microbes throughout the years, some without the protection of antibodies or immune effector cells [7]. On a daily basis we are exposed to a number of pathogens through ingestion or contact with infected surfaces [8]. The reason we continue to survive is a non-specific broad spectrum response to injury and infection created by our innate immune response [9]. All organisms possess a variety of broad-spectrum antimicrobial peptides known as cationic peptides [7]. Microbial pathogens don’t seem to acquire resistance to these cationic peptides even though they have often been exposed to them for a very long time [7]. For this reason, there has been a lot of commercial interest in and effort put into developing cationic peptides as potential antimicrobial therapeutics [7].
Cationic peptides
Approximately 20 years ago, it was discovered that the skin of frogs, the lymph of insects, and human neutrophils (a type of white blood cell) all contain cationic peptides that can act as potent antimicrobials [3]. To date more than 600 cationic peptides have been discovered in virtually all organisms from microbe to man. These cationic peptides have a broad spectrum of antimicrobial activity, including activity against bacteria, eukaryotic parasites, viruses, and fungi [3]. Rarely are the same cationic peptide sequences recovered from different species, even those that are closely related [7]. Cationic peptides vary considerably in sequence and structure, with a few common features [10,11]. All cationic peptides are encoded as larger precursors with signal sequences that are subsequently modified by cleavage or group addition reactions such as glycosylation or halogenation [7]. They range in size from 12-50 amino acids and have molecular masses of less than 10000 [3,6]. Cationic peptides are amphipathic meaning they possess both a hydrophobic region that interacts with lipids and a positively charged hydrophilic region that interacts with water or negatively charged residues [3,6]. This feature allows the peptides to interact well with membranes that are composed of amphipathic molecules, especially negatively charged bacterial membranes [3]. For the most part, animal cells tend to have membranes with no net charge so they are unaffected by cationic peptides [6]. There are four major classes of cationic peptides [3]:
- β-sheet molecules stabilized by two or three disulphide bonds
- amphipathic α-helices
- extended molecules
- loops due to a single disulphide bond
Cationic peptides have been found to have activity against both Gram negative and Gram positive bacteria as well as fungi, eukaryotic parasites, and viruses [3]. Most importantly, cationic peptides are effective against strains of antibiotic resistant bacteria [3].
Mechanisms of action
Cationic peptides interact with bacterial membranes3. Bacterial cells may have two membranes on which cationic peptides can act:
- the outer membrane
- the cytoplasmic membrane
.thumbnail.gif)
1. Action of cationic peptides on outer membrane
In Gram negative bacteria, it is hypothesized that cationic peptides interact with the highly negatively charged outer membrane. The surface of the membrane contains magnesium ions which function to neutralize the charge and it is believed that the cationic peptides displace these magnesium ions [3]. The peptides then either bind tightly to the negatively charged membrane lipopolysaccharide (LPS) or neutralize the charge over an area of membrane subsequently distorting the membrane structure [3]. Once this occurs the peptides can translocate across the outer membrane.
2. Action of cationic peptides on cytoplasmic membrane
The bacterial cytoplasmic membrane is also negatively charged. Cationic peptides can insert in a position parallel to the membrane lipids and fold into membrane-bound structures leading to four possible outcomes [3]:
- cell lysis, although this is not very likely at physiological concentrations of cationic peptide
- channel formation, where the peptides reorient themselves in the cytoplasmic membrane in such a way that they form channels leading to a breakdown in membrane integrity
- the carpet model where cationic peptides cause the catastrophic breakdown of the membrane integrity
- direct killing of the bacterial cell by peptides attacking internal targets like cellular polyanions (for example, nucleic acids) in the cytoplasm after diffusing through both membranes
Roles in immunity
Cationic peptides not only directly kill bacteria, they also have profound effects on the host system [8,12-14]. It has been shown that cationic peptides can function to sequester LPS shed from Gram negative bacteria. This LPS functions as an endotoxin potentially leading to sepsis [3]. Cationic peptides have also been found to be potent chemo-attractants, recruiting antigen-presenting cells, which can initiate responses by the adaptive immune system [15]. They have also been known to recruit mast cells (a type of white blood cell), which are known to increase blood vessel permeability in order to allow other immune effectors and wound healing factors to permeate injured tissues [15].
Later, at the clinic
In order to be a good candidate for therapeutic use, a drug must show good activity, appropriate function, low toxicity, have stability in vivo and be reasonably inexpensive to manufacture [3]. So far only a few cationic peptides have made their way to clinical trials and only two are used in topical creams and solutions [3]. One of the problems that limits the systemic use of these compounds is toxicity [3]. Currently research is being done to structurally alter these compounds to make them less toxic. Another problem associated with administering cationic peptides as a treatment for infection is the inability to direct the peptides to appropriate locations with accuracy [6]. When injected intravenously the peptides are required to infiltrate healthy tissue in order to reach the appropriate locations, which can be a very slow process [6]. The host’s system can also act on the peptides; for example, the presence of proteases can inactivate peptides before they reach their destination [3]. Finally, sometimes the activities of the peptides are not the same in vitro and in vivo. For example one of the most potent cationic peptides studied to date in vitro, polyphemusin I from horseshoe crabs, has been shown to have no activity against infections in animal models [16]. Finally, cationic peptides are also very expensive to produce because they cannot be mass-produced in bacteria or fungi the way many other antimicrobial drugs are produced. Consequently, the cost of treatment is estimated at approximately $100 a day [3].
Applications in biotech
Cationic peptides have a number of applications in biotechnology. One application for cationic peptides is the formation of transgenic plants. Phytopathogens are a major problem in cultivated and stored crops leading to the loss of between 30-50 billion dollars annually [17]. Crop spoilage also increases carcinogen incidence and has adverse affects on human health [18]. Pesticides and antimicrobials are not really a good way to combat phytopathogens because they can be expensive and detrimental to the environment and to humans [18]. They also can lead to the development of resistant bacterial strains. It is hypothesized that by producing transgenic plants that express cationic peptides with broad specificity many of these problems will be circumvented [18]. Preliminary results from the University of Victoria indicate that this approach may be useful18. Microgene International Ltd. is currently using the technology to engineer genetically enhanced fish which over-produce cationic peptides [19]. The hope is that these fish will not be as susceptible to infections. Normally 25% of aquaculture stock are susceptible to infections and consequently lost [19]. Another potential application is the formation of aerosol sprays for patients with cystic fibrosis [19]. Cystic fibrosis is a disease caused by a genetic mutation in the CFTR gene. This mutation leads to the inability to regulate chloride ion concentrations across membranes. The build up of high chloride salt concentration in the lungs reduces the CF patients’ cationic peptide function [19]. These individuals then become more susceptible to infections by opportunistic bacteria such as Pseudomonas aeruginosa [19]. Through molecular biological techniques it may be possible to engineer cationic peptides in such a way that they will be less susceptible to high salt concentrations [19].
Conclusion
As bacteria are becoming resistant to conventional antibiotics researchers are looking for new ways to combat microbial infections. One possibility is the development of cationic peptides as commercial antimicrobial agents. Cationic peptides are present in all organisms and function in the killing of bacteria, viruses, eukaryotic parasites, and fungi. Bacteria don’t seem to acquire resistance to cationic peptides, which make them an attractive model for drug companies. Another plus is that they are rarely the same in different organisms, which could potentially lead to a greater selection of drugs. They may also be useful in other biotechnological applications in terms of transgenically altering plants and animals in agriculture with cationic peptides to reduce losses. There are still many barriers to the development of cationic peptides as commercial antimicrobial agents but, with time and future research, perhaps they may provide a feasible option for managing microbial infections.
Glossary
- Glycosylation- The addition of carbohydrate (sugar) groups to a molecule, especially a pre-formed polypeptide
- Halogenation – The addition of halogen (F, Cl, Br, I, At) groups to a molecule, especially a pre-formed polypeptide
- β-sheet – A sheet-like secondary structure protein motif resulting from hydrogen bonding between adjacent beta strand regions
- α-helices – A helical secondary structure protein motif placing all the functional groups of amino acids on the outside
- Extended molecules -Proteinaceous particles lacking typical motifs.
- Disulphide bond – A covalent interaction between two polypeptide strands wherein the sulfurs of two cysteine residues oxidize to form a double bond
- Phytopathogen – An organism that benefits from an interaction with a plant, and has a negative effect on that plant
References
1. Edinburgh, T. U. o. http://helios.bto.ed.ac.uk/bto/microbes/penicill.htm.
2. Hancock, R. & Knowles, D. Are we approaching the end of the antibiotic era? Current opinion in Microbiology 1, 493-494 (1998).
3. Hancock, R. E. Cationic peptides: effectors in innate immunity and novel antimicrobials. Lancet Infect Dis 1, 156-64 (2001).
4. Breithaupt, H. The new antibiotics. Nat Biotechnol 17, 1165-9 (1999).
5. Davies, J. Inactivation of antibiotics and the dissemination of resistance genes. Science 264, 375-82 (1994).
6. Ganz, T. Chemistry. Rings of destruction. Nature 412, 392-3 (2001).
7. Zasloff, M. Antimicrobial peptides of multicellular organisms. Nature 415, 389-95 (2002).
8. Hancock, R. E. & Diamond, G. The role of cationic antimicrobial peptides in innate host defences. Trends Microbiol 8, 402-10 (2000).
9. Janeway, C. A., Jr. Presidential Address to The American Association of Immunologists. The road less traveled by: the role of innate immunity in the adaptive immune response. J Immunol 161, 539-44 (1998).
10. Hancock, R. E. Cationic antimicrobial peptides: towards clinical applications. Expert Opin Investig Drugs 9, 1723-9 (2000).
11.Ganz, T. & Lehrer, R. I. Antimicrobial peptides of vertebrates. Curr Opin Immunol 10, 41-4 (1998).
12. Scott, M. G. & Hancock, R. E. Cationic antimicrobial peptides and their multifunctional role in the immune system. Crit Rev Immunol 20, 407-31 (2000).
13. Boman, H. G. Peptide antibiotics and their role in innate immunity. Annu Rev Immunol 13, 61-92 (1995).
14. Gudmundsson, G. H. & Agerberth, B. Neutrophil antibacterial peptides, multifunctional effector molecules in the mammalian immune system. J Immunol Methods 232, 45-54 (1999).
15. Ganz, T. Defensins and host defense. Science 286, 420-1 (1999).
16. Zhang, L., Scott, M. G., Yan, H., Mayer, L. D. & Hancock, R. E. Interaction of polyphemusin I and structural analogs with bacterial membranes, lipopolysaccharide, and lipid monolayers. Biochemistry 39, 14504-14 (2000).
17. Baker, B., Zambryski, P., Staskawicz, B. & Dinesh-Kumar, S. P. Signaling in plant-microbe interactions. Science 276, 726-33 (1997).
18. Osusky, M. et al. Transgenic plants expressing cationic peptide chimeras exhibit broad-spectrum resistance to phytopathogens. Nat Biotechnol 18, 1162-6 (2000).
19 www.nce.gc.ca. (1997).
(Art by Jen Philpot – note that higher res versions of these image files are available here)